Lab Experimental Characterization of Smart Antenna / MIMO Systems
The increasing demand for high-speed, high-quality mobile communication has fueled interest in spectrum-efficient multiple-input multiple-output (MIMO) wireless communication links. Systems with MIMO communication links use multiple antenna arrays, one at the transmitter and one at the receiver, to take full advantage of the spatial dimension of the propagation channel. When properly designed, multi-array communication links can provide multi-fold increases in link throughput in addition to dramatic reductions in channel variation. This can be used to provide higher data rates to single users, lower delay links, or to allow multiple users to coexist in the spatial channel. Due to these advantages, MIMO capability is being considered for indoor local area networks, cellular multimedia data networks, and broadband fixed wireless access.
Orthogonal frequency-division multiplexing (OFDM) is a modulation technique designed for frequency selective communication mediums that does not increase the complexity of equalization at the receiver and allows for high throughputs. OFDM divides the wideband, frequency selective transmission channel into several orthogonal, flat fading narrowband channels. Thus, each sub-frequency can be treated as an independent channel, allowing for various forms of adaptive modulation techniques to be performed on each subcarrier.
Adaptive Bit Loading
One such adaptive technique is Adaptive Bit Loading (ABL), which aims to increase spectral efficiency of the communication link while subjected to power and/or bit error rate (BER) constraints. ABL related research at DWSL has primarily focused on Rate-Adaptive Bit Loading, which strives to increase overall data rates in the wireless link without increasing overall transmit power and while subjected to a desired BER threshold. This is accomplished through optimization algorithms that, in essence, transmit higher data rates on stronger OFDM subcarriers and lower data rates on weaker subcarriers. Research at DWSL has indicated that a sub-optimal form of this adaptive technique shows the greatest success in slow fading, highly frequency channels. Additional research areas in wireless OFDM ABL also include the reduction of training feedback overhead and compensation for channel estimation inaccuracies.
Peak-to-Average Power Ratio
On the other hand, one of the main disadvantages of OFDM is its high Peak to Average Power Ratio (PAPR). High signal peaks cause amplifiers to operate in non-linear regions, which may result in non-linear intermodulation distortion and out-of-band radiation. In some implementations where the signal to be transmitted is normalized with respect to its peaks, high PAPR can reduce transmission range. At DWSL, we have focused on the latter problem and have evaluated through simulations and measurements how algorithms that aim to mitigate PAPR actually benefit the system by improving transmit power. The post processing signal to noise ratio (PPSNR) is the metric used to evaluate these algorithms. Figures 1 and 2 are examples of how the PPSNR can improve when sequences with small PAPR are selected for transmission. Here, PAPR reduction is performed by first interleaving frequency domain symbols and selecting those sequences with minimal PAPR.
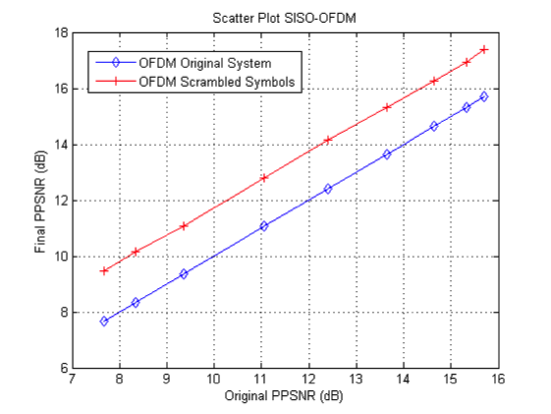
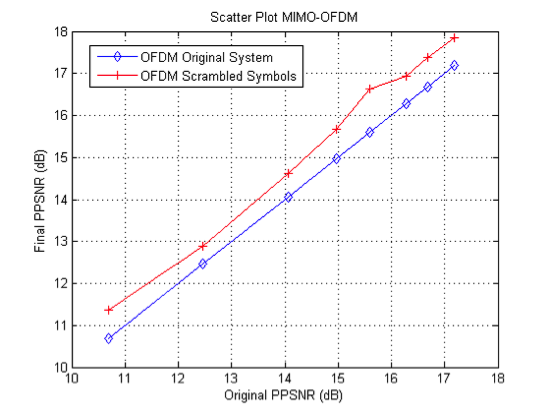
Another branch of research currently being developed by DWSL joins PAPR reduction and ABL to define a new rate adaptive and symbol rotation scheme capable of achieving higher throughputs with increased reliability through PAPR reduction
Measurement Capabilities
The theoretical capabilities of MIMO have generated a tremendous amount of interest in the communications literature. However, the practical considerations of implementing such communication links must be considered before any systems are deployed. For example, one critical assumption in much of the work on MIMO systems is that scattering in the propagation channel decouples the elements of the antenna arrays. In real systems, however, the antenna elements are mutually coupled due to complex interaction between antenna elements and other conductors in the near-field of the antenna. Without careful design, the results of electromagnetic interaction, such as mutual coupling, may correlate the channel coefficients thus resulting in a loss in terms of capacity or error probability.
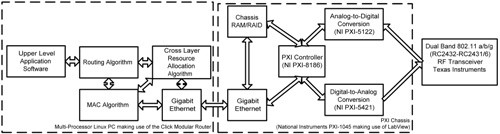
To facilitate the study of the electromagnetic interactions in wideband MIMO communication links, a multi-component measurement apparatus is being constructed. The system will consist of 802.11a/b/g transceivers (donated by Texas Instruments) to implement the RF front end, and high-speed sampling boards and arbitrary waveform generator boards (donated by National Instruments) to implement the baseband. Each node currently has 2 RF antenna array elements and transceivers, and the system can be expanded without re-design to 4 array elements and transceivers.
In each node, there are three main components, an analog front end / transceiver that can include conformal or standalone antenna arrays, a frequency agile RF transceiver donated from Texas Instruments (RCS 110), a software defined baseband data acquisition system (National Instruments PXI 5122 and 5421), and general purpose PCs for the MAC and higher layers. The RF transceiver cards are capable of transmitting at all channels in both the Industrial, Science, and Medicine (ISM) band between 2.4 and 2.5 GHz as well as in the Unlicensed National Information Infrastructure (UNII) band between 4.9 and 5.9 GHz. The cards are designed to operate independently of existing wireless standards, and thus provide us with maximum flexibility.
The analog front end and the baseband data acquisition system are all contained in a PXI chassis from National Instruments. Analog signals are converted to and from digital using PXI data acquisition cards from National Instruments and then passed to a dedicated chassis processor (PXI-8186) which implements the physical layer in LabView. This processor then communicates over gigabit ethernet with a general purpose host which runs the MAC, higher layer network protocols, and any application software.
The testbed is currently be used to facilitate a wide variety of undergraduate and graduate research projects including MIMO-OFDM channel and interference sounding, reconfigurable antennas, antenna array design and fabrication, game-theoretic resource allocation algorithms, MIMO-aware LAN algorithms, and cross-modality networking.
DWSL also owns 5 MIMO nodes of the Wireless Open-Access Resarch Platform (WARP) developed by Rice University. Baseband processing in WARP is achieved using a Xilinx Virtex-II PRO FPGA capable of processing data at 100s of Megabits per second with onboard embedded PowerPC processor cores. Each WARP node daughtercard has ISM and UNII band radio transmitters with dual 65 MS/sec 14-bit ADCs and dual 125 MS/sec 16-bit DACs. This platform is flexible, allowing for software reconfigurability of all layers of the protocol stack. WARP is also modular so that new daughtercard modules can be implemented to prototype communication networks with other frequency bands, radio standards, or communication modalities (e.g., optical, ultrasound). Programming WARP can be accomplished using a variety of interfaces ranging from low level VHDL code, Xilinx System Generator, and Matlab for physical layer algorithm development and Platform Studio in C/C++ for implementing higher layers of the protocol stack. Furthermore, WARP has a large community of users to provide technical support and a vehicle for dissemination of research results and newly developed communication and networking techniques.